Chytrid batrachochytrium salamanderdivorans
Batrachochytrium salamandrivorans sp. nov. causes lethal chytridiomycosis in amphibians
An Martela,1, Annemarieke Spitzen-van der Sluijsb, Mark Blooia, Wim Bertc, Richard Ducatellea, Matthew C. Fisherd, Antonius Woeltjesb, Wilbert Bosmanb, Koen Chiersa, Franky Bossuyte, and Frank Pasmans. Department of Pathology, Bacteriology and Avian Diseases, Faculty of Veterinary Medicine, Ghent University, B-9820 Merelbeke, Belgium; bReptile, Amphibian and Fish Conservation The Netherlands, 6501 BK, Nijmegen, Netherlands; cDepartment of Biology, Nematology Unit, Faculty of Science, Ghent University, 9000 Ghent, Belgium; dDepartment of Infectious Disease Epidemiology, Faculty of Medicine, Imperial College London, London W2 1PG, United Kingdom; and eAmphibian Evolution Lab, Biology Department, Vrije Universiteit Brussel, 1050 Brussels, Belgium Edited by David B. Wake, University of California, Berkeley, CA, and approved August 1, 2013 (received for review April 18, 2013)
The current biodiversity crisis encompasses a sixth mass extinction event affecting the entire class of amphibians. The infectious disease chytridiomycosis is considered one of the major drivers of global amphibian population decline and extinction and is thought to be caused by a single species of aquatic fungus, Batrachochytrium dendrobatidis. However, several amphibian population declines remain unexplained, among them a steep decrease in fire salamander populations (Salamandra salamandra) that has brought this species to the edge of local extinction. Here we isolated and characterized a unique chytrid fungus, Batrachochytrium salamandrivorans sp. nov., from this salamander population. This chytrid causes erosive skin disease and rapid mortality in experimentally infected fire salamanders and was present in skin lesions of salamanders found dead during the decline event. Together with the closely related B. dendrobatidis, this taxon forms a well-supported chytridiomycete clade, adapted to vertebrate hosts and highly pathogenic to amphibians. However, the lower thermal growth preference of B. salamandrivorans, compared with B. dendrobatidis, and resistance of midwife toads (Alytes obstetricans) to experimental infection with B. salamandrivorans suggest differential niche occupation of the two chytrid fungi. amphibian decline | emerging infectious disease | ecosystem health
Amphibians have become an icon of the global biodiversity crisis (1). Although a variety of factors are involved in amphibian decline worldwide, fungal chytridiomycosis has been identified as one of the major infectious diseases involved, resulting in the extirpation of >40% of amphibian species in areas in Central America and widespread losses across Europe, Australia, and North America (2, 3). Chytridiomycosis is currently considered to be caused by a single species of fungus, Batrachochytrium dendrobatidis, which is the only chytridiomycete taxon known to parasitize vertebrate hosts. However, B. dendrobatidis and other factors known to cause amphibian decline fail to explain several recent amphibian population losses (4, 5).
A dramatic and enigmatic mortality event, which has brought this species to the edge of extinction, was recently reported among fire salamanders (Salamandra salamandra) in The Netherlands (5). Since 2010, the species has declined, with only 4% of the population remaining in 2013. This rapid decline coincided with the finding of dead animals in the field (5). The recent startup of an ex situ conservation program for 39 of the remaining fire salamanders was compromised by the unexplained death of 49% of the captive animals between November and December 2012. Attempts to identify known amphibian infectious agents, including B. dendrobatidis, in these salamanders yielded negative results (5). Instead, we found, isolated, and characterized a second, highly pathogenic chytrid fungus from this decline event that occupies a different niche compared with B. dendrobatidis.
Results and Discussion
The chytrid fungus was isolated from the skin of fire salamanders from the affected population in Bunderbos (N50°54′51″, E5°44′
www.pnas.org/cgi/doi/10.1073/pnas.1307356110
59″), The Netherlands. Phylogenetic analyses including a broad range of representative chytrid species show that this fungus represents a previously undescribed lineage that forms a clade with B. dendrobatidis (Fig. 1; Table S1). Its considerable genetic distance from B. dendrobatidis (3.47–4.47% for the 1,513 18S + 28S rRNA base pairs) compared with the shallow divergences between B. dendrobatidis isolates (6) warrants the description of a unique species within the chytridiomycote order Rhizophydiales (family incertae sedis): Batrachochytrium salamandrivorans spec. nov. The unique chytrid represented by isolate AMFP13/1 (the holotype in liquid nitrogen at Ghent University) is the second chytrid known to parasitize and kill amphibians. In vitro, the unique taxon produces motile zoospores, which emerge from colonial (a single thallus containing multiple, walled sporangia) or monocentric thalli (Fig. 2A). The most obvious morphological differences, compared with the B. dendrobatidis type strain, are the formation of germ tubes in vitro (Fig. 2B; Fig. S1) and the abundant formation of colonial thalli both in vitro and in vivo (Fig. 3B). B. salamandrivorans grew at temperatures as low as 5 °C, with optimal growth between 10 °C and 15 °C and death at ≥ 25 °C, a markedly lower thermal preference compared with B. dendrobatidis (7) (Fig. 4).
Significance
Chytridiomycosis has resulted in the serious decline and extinction of >200 species of amphibians worldwide and poses the greatest threat to biodiversity of any known disease. This fungal disease is currently known to be caused by Batrachochytrium dendrobatidis, hitherto the only species within the entire phylum of the Chytridiomycota known to parasitize vertebrate hosts. We describe the discovery of a second highly divergent, chytrid pathogen, Batrachochytrium salamandrivorans sp. nov., that causes lethal skin infections in salamanders, which has resulted in steep declines in salamander populations in northwestern Europe. Our finding provides another explanation for the phenomenon of amphibian biodiversity loss that is emblematic of the current global biodiversity crisis.
Author contributions: A.M. and F.P. designed research; A.M., M.B., and F.P. performed research; A.M., A.S.-v.d.S., W.B., R.D., M.C.F., A.W., W.B., K.C., and F.P. contributed new reagents/analytic tools; A.M., F.B., and F.P. analyzed data; A.M., M.C.F., F.B., and F.P. wrote the paper; A.S.-v.d.S., A.W., and W.B. contributed field data; R.D. and K.C. performed histopathology; M.C.F. delivered DNA and genetic data; F.B. performed phylogenetic analysis; and A.M. and F.P. discovered the fungus.
The authors declare no conflict of interest.
This article is a PNAS Direct Submission.
Freely available online through the PNAS open access option.
Data deposition: The sequences reported in this paper have been deposited in the GenBank database (accession nos. KC762294, KC762293, and KC762295), and the description of the fungus has been deposited in MycoBank (accession no. MB803904).
Fig. 1. Maximum likelihood tree (−Ln L = 9,562.04266) for the analysis of a 1,513-bp data matrix of partial 18S + 28S rRNA genes. Together with B. dendrobatidis, B. salamandrivorans sp. nov. forms a well-supported clade [maximum parsimony bootstrap support = 100; maximum likelihood bootstrap support (MLBS) = 100; Bayesian posterior probability (BPP) = 100] of Chytridiomycota that parasitize amphibians with potentially lethal consequences. Squares on branches indicate MLBS > 70 and BPP > 95; triangles indicate MLBS < 70 and BPP > 95.
Infected fire salamanders died within 7 d after a short episode of anorexia, apathy, and ataxia. The pathology consistently comprised multifocal superficial erosions and deep ulcerations in the skin all over the body. Keratinocytes with eosinophilic necrosis and marginated nuclei were at the periphery of the erosions. Each of these keratinocytes contained one centrally located thallus, the majority being segmented (colonial thalli). Bacteria superficially colonized the ulcers. Additionally, anywhere in the skin, small foci of keratinocytes immediately below the damaged keratin layer were found. These presented similar eosinophilic necrosis, marginated nuclei, and centrally located colonial thalli. The intraepidermal organisms did stain with immunohistochemistry (8) (Fig. 3A). Transmission electron microscopic examination of the skin lesions confirmed the presence of intracellular structures consistent with the colonial thalli (Fig. 3B). All animals were also screened for a wide array of other infectious diseases, but no evidence for any other pathology was found: neither PCR (9) nor quantitative PCR (qPCR) (10) suggested the presence of chytrid B. dendrobatidis DNA in the skin samples. Virological examination [including PCR for the detection of herpes viruses (11), adenoviruses (12), and ranaviruses (13) and inoculation of IgH2 (iguana heart epithelial cells) and RTG (rainbow trout gill) cell cultures for general virological investigation] was negative. Ziehl Neelsen staining, PCR for Chlamydiaceae (14), and bacterial isolation attempts did not yield any evidence of bacterial infections.
To further demonstrate that salamandrid mortality was caused by B. salamandrivorans, we performed infection experiments on healthy fire salamanders (n = 5) by exposing them to 5,000 zoospores of B. salamandrivorans for 24 h. All animals died 12– 18 d after inoculation after a 1- to 2-d episode of ataxia. Isolation was attempted and succeeded from one deceased salamander. PCR (described below) showed that B. salamandrivorans DNA was present in all five infected animals, coinciding with histopathological lesions consisting of focal epidermal ulceration with very high numbers of colonial thalli of B. salamandrivorans, which matched the lesions found in wild animals. B. salamandrivorans– induced lesions are characterized by marked skin ulceration, opposed to those caused by B. dendrobatidis, which typically induces epidermal hyperplasia and hyperkeratosis (15). No clinical signs or histopathological lesions were observed in the uninfected negative control animals (n = 5). Additionally, we put two healthy fire salamanders in a terrarium with an infected individual for 2 d. One salamander died 22 d after contact and the other 27 d after being placed with the infected animal. Histology, immunohistochemistry
(8), and PCR demonstrated the presence of high numbers of
B. salamandrivorans in their epidermal layers, with lesions identical to those described above. Cohousing on damp toweling effectively transmitted B. salamandrivorans and caused death in <1 mo. Experimentally infected midwife toads (Alytes obstetricans), the species that is most highly susceptible to infection by B. dendrobatidis in Europe (16, 17), did not show any signs of colonization by B. salamandrivorans, as determined by immunohistochemistry and PCR, or disease, suggesting a differential amphibian host range for the two chytrids.
Amphibians will clearly benefit from the rapid identification of areas in which B. salamandrivorans is present. We therefore designed diagnostic species-specific PCR primers to amplify the 5.8S ribosomal RNA gene and its flanking internal transcribed spacer regions: ITS1 and ITS2. Our set of primers STerF and STerR amplified B. salamandrivorans in all positive tissues examined. Importantly, these primers did not amplify any of the nine tested strains from all three B. dendrobatidis lineages known to infect Europe and therefore provide a rapid noninvasive method for detecting of B. salamandrivorans infections. Furthermore, by using the newly developed PCR primers, we were also able to detect B. salamandrivorans DNA in remains of the epidermises of six wild fire salamanders (from Bunderbos, The Netherlands) that were found dead in 2010 or 2011 and were stored at −70 °C. B. salamandrivorans was found present in skin swabs from all five experimentally infected and moribund fire salamanders, but in none of the midwife toads and noninfected
2 of 5 | www.pnas.org/cgi/doi/10.1073/pnas.1307356110 Martel et al.
Fig. 2. In vitro culture of B. salamandrivorans in TGhL broth at 15 °C. (A) Monocentric thalli predominate, with the rare presence of colonial thalli (black arrow). Sporangia develop discharge tubes (white arrow) to release zoospores (Scale bar, 100 μm.) (B) Scanning electron microscopic image of a mature sporangium with rhizoids (R), discharge tubes (D), and germ tube formation (arrow) (Scale bar, 10 μm.)
Fig. 3. Microscopy of the skin of a fire salamander that died due to infection with B. salamandrivorans. (A) Immunohistochemical staining of a 5-μm skin section. Intracellular colonial thalli abound throughout all epidermal cell layers and are associated with erosive lesions. (Scale bar, 20 μm.) (B) Transmission electron microscopy picture of an intracellular colonial thallus of B. salamandrivorans inside a keratinocyte (Scale bar, 4 μm.)
fire salamanders. Additionally, 13 of 33 swabs collected from live fire salamanders from the declining population in Bunderbos, The Netherlands, in 2010 tested positive with this PCR, in contrast to 0 of 51 swabs from fire salamanders from a stable population in Belgium. Our PCR method thus allows the rapid screening of both extant populations and archived specimens for the presence of B. salamandrivorans–induced chytridiomycosis. Chytridiomycosis in amphibians can no longer be attributed to a single species of chytrid, but can be caused by either B. dendrobatidis or B. salamandrivorans. Our results reveal striking similarities and differences between B. salamandrivorans and the behavior of the hypervirulent global pandemic lineage of B. dendrobatidis (18). Both fungal species share at least the following hallmarks: (i) induction of a lethal skin disease and (ii) association with mortality events and severe population decline. In contrast, it is as yet unclear to what extent B. salamandrivorans is capable of infecting a broad amphibian host range, as is the case for B. dendrobatidis (3). However, development of erosive vs. hyperplastic/hyperkeratotic skin lesions, failure to experimentally infect midwife toads, and relatively low thermal preferences of B. salamandrivorans suggest differential host specificity of the two pathogens and possibly a differential effect on amphibian assemblages. Because the majority of recent B. dendrobatidis surveillance worldwide is based on the B. dendrobatidis–specific qPCR (10), it is currently impossible to estimate the extent and impact of B. salamandrivorans on amphibian populations worldwide using the B. dendrobatidis mapping framework (19). However, the emergence of the pathogenic B. salamandrivorans chytrid fungus is worrying and warrants close monitoring, urgent risk analysis, and its inclusion in any monitoring program assessing amphibian population health.
Taxonomy. Batrachochytrium salamandrivorans Martel, Blooi, Bossuyt and Pasmans sp. nov. MycoBank accession no. MB803904.
In vitro (tryptone-gelatin hydrolysate-lactose broth). Thalli predominantly monocentric, although some colonial. Development exogenous with sporangia forming at tip of germ tube. Rhizoids fine, isodiametric, extending from a single or several areas, lacking subsporangial swelling; Sporangium diameter 15.7–50.3 μm (average, 27.9 μm). One to several discharge papillae; cell wall at tip discharge papillae forms plugs that deliquesce resulting in release
Martel et al.
of motile zoospores. Motile zoospores roughly spherical, with highly irregular surface and cell surface projections; diameter 4.0– 5.5 μm (average 4.6 μm). Resting spore not observed. Growth at 5, 10, 15, 20, and 22 °C, but not at temperatures ≥24 °C. Death of thalli after 5 d at 25 °C. Five-day generation time at 15 °C. In Vivo. In epidermis of amphibians; forming predominantly colonial thalli that contain several walled sporangia. Thalli located inside keratinocytes; diameter 6.9–17.2 μm (average 12.2 ± 1.9 μm, n = 50).
Zoospore Ultrastructure. Ultrastructure highly similar to that of B. dendrobatidis. Nucleus located outside the ribosomal mass, multiple mitochondria and numerous lipid globules. Position of the nonflagellated centriole in free swimming zoospores varies from angled to parallel to kinetosome. rDNA Sequences. Partial nucSSU rDNA GenBank accession no. KC762294, partial nucLSU rDNA GenBank accession no. KC762293, partial ITS1-5.8S-ITS2 rDNA GenBank accession no. KC762295.
Holotype. Isolate AMFP13/1 (CBS 135744) from a fire salamander (Salamandra salamandra), kept in liquid nitrogen at Ghent
University.
Etymology. The species epithet salamandrivorans (sa.la.man.dri. vo’rans. L. n. salamandra, salamander; L. part. adj. vorans, eating, devouring; N.L.part. adj. salamandrivorans, salamander-devouring) refers to the extensive skin destruction and rapid mortality observed in infected salamanders.
Materials and Methods
Postmortem Examination of Fire Salamanders. Six S. salamandra that died in captivity between November and December 2012 were subject to gross necropsy, histopathology, and routine bacteriological, mycological, and virological examinations. Histological examination of liver, spleen, kidney, lung, gonad, midgut, and skin was done using microscopic examination of paraffin-embedded, 5-μm tissue sections stained with H&E, Ziehl Neelsen, or periodic acid shift. A 1:10 (vol:vol) tissue suspension of these organs in PBS was inoculated on sheep blood and tryptic soy agar and incubated at 20 °C and 30 °C. A liver suspension was inoculated on IgH2 and RTG cells. PCRs were performed to detect the presence of herpesviruses (11), adenoviruses (12), iridoviruses (13), Chlamydiales (14), and B. dendrobatidis (9, 10). Immunohistochemistry was performed on all skin samples to detect B. dendrobatidis antigens (8). Transmission electron microscopy of epidermal samples was performed with glutaraldehyde fixation in 0.05 M sodium cacodylate buffer,
Fig. 4. Growth of B. salamandrivorans in TGhL broth at different temperatures. (A) Growth was quantified by calculating the average percentage ± SD of the surface area of three wells covered by the fungus after 10 d of incubation at a given temperature. Motile zoospores were present at 5–20 °C, but not at 22, 24, and 30 °C. (B) B. salamandrivorans growth after 10 d at 4 °C
(a), 15 °C (b), 20 °C (c), and 30 °C (d) (Scale bar, 200 μm.)
1% osmium tetroxide postfixation, and en bloc staining for 1 h in a 1% solution of uranyl acetate. Five S. salamandra specimens were found dead in the field during 2010 and 2011. Due to the severe autolysis of these animals, the postmortem examination was limited to skin histopathology and PCR for the detection of herpesviruses, adenoviruses, iridoviruses, Chlamydiaceae, and B. dendrobatidis.
B. salamandrivorans Strain Isolation and Culture Conditions. Chytrid isolation on tryptone-gelatin hydrolysate-lactose (TGhL) agar plates containing penicillin/streptomycin (200 mg/L) at 20 °C was attempted from the dead S. salamandra as described previously for the isolation of B. dendrobatidis (7). Skin samples without contaminating bacterial or fungal growth were transferred to TGhL broth once zoospores were seen on the agar plates. The isolate was subsequently subcultured in TGhL broth in cell culture flasks at 15–20 °C. A 10-d-old subculture was frozen in liquid nitrogen (20). To obtain zoospores, 1 mL of a culture growing in TGhL broth was transferred to a TGhL agar plate and incubated for 5–10 d at 15 °C. Zoospores were obtained by washing the agar plate with 2 mL of 0.2-μm filtered pond water. The number of zoospores in the suspension was determined using a hemocytometer.
To determine thermal growth conditions, 200 μL of a 5-d-old
B. salamandrivorans culture in TGhL broth at 15 °C was transferred to the wells of a 24-well plate, and 0.8 mL of TGhL broth was added. The plates were incubated at 5 °C, 10 °C, 15 °C, 20 °C, 22 °C, 23 °C, 24 °C, 25 °C, and/or 30 °C ± 1 °C for 10 d. Growth was defined as a significant increase of the surface of the well covered by the fungus compared with wells incubated at 30 °C (which is above the lethal temperature for B. salamandrivorans) and the presence of motile zoospores. The surface coverage was determined by image analysis (GNU Image Manipulation Program) of pictures, taken through an inverted light microscope (Nikon Eclipse ts100, 20× magnification). Each condition was tested in triplicate. If no growth was seen after 10 d of incubation, the plates were further incubated at 15 °C. Cultures were considered dead if no growth occurred within 10 d. B. salamandrivorans Molecular Characterization and Diagnostic PCR Development. PCRs were done on the chytrid culture obtained to amplify the 18S, 28S, and the 5.8S rRNA genes and the flanking ITS regions ITS1 and ITS2 (21). Based on the ITS1-5.8S-ITS2 sequence, the primer set (STerF 5′TGCTCCATCTCCCCCTCTTCA3′ and STerR 5′TGAACGCACATTGCACTCTAC3′) was developed and used to detect the 5.8S rRNA gene of B. salamandrivorans in skin samples from the six S. salamandra found dead in the field, six animals that died in captivity, and 33 swabs collected from S. salamandra in Bunderbos in 2010. Amplification reactions consisted of 10 ng DNA, 1 μM of each primer, 1.5 mM MgCl2, 1× Taq buffer, 0.2 mM of each dNTP, and 0.8 units of Taq polymerase in a volume of 20 μL. PCR amplification was performed under the following conditions: 10 min at 93 °C, followed by 30 cycles of 45 s at 93 °C, 45 s at 59 °C, 60 s at 72 °C, and 10 min at 72 °C. DNA of a pure culture of B. salamandrivorans was used as a positive control. Using primer set STerF and STeR, we assessed whether DNA of nine B. dendrobatidis strains would be amplified—Cape lineage (BdCAPE) isolates: SA1D, TF5a1, and CCB1; Swiss lineage (BdCH) isolates: Con2A, APEP, and 0739; and the global panzootic lineage (BdGPL) isolates: MAD, IA042, and JEL197. All derived amplicons were sequenced.
Phenotypic Characterization. The morphology of the chytrid isolate in TGhL agar and broth was examined using inverted, phase contrast, and scanning (22) and transmission electron microscopy (23). Zoospores were collected from growth on TGhL agar plates and fixed for transmission electron microscopy with s-collidine buffer followed by osmium tetroxide (23).
Experimental Infection of Fire Salamanders and Midwife Toads. The animal experiment was performed with the approval of the ethical committee of the Faculty of Veterinary Medicine (Ghent University, EC2013/10) under strict BSL2 conditions. Ten captive bred fire salamanders (S. salamandra) and midwife toads (Alytes obstetricans) were housed individually at 15 ± 1 °C on moist tissue, with access to a hiding place and a water container. All animals were clinically healthy and free of B. dendrobatidis as assessed by sampling the skin using cotton-tipped swabs and subsequent performing qPCR (10). Using the PCR described above, all swab samples were negative for the presence of DNA of B. salamandrivorans. After 1 wk of acclimatization, 1 mL of a zoospore suspension in filtered (0.2 μm) pond water, containing 5,000 zoospores/mL, was dripped on the five animals of each species. Animals were fed twice weekly with crickets and followed up by clinical examination and weekly collection of skin swabs until 3 wk after exposure. The skin swabs were examined for the presence of B. salamandrivorans DNA as described elsewhere.
Skin Swabs from Declining and Stable S. salamandra Populations. Skin swabs were collected from 33 S. salamandra from the Dutch fire salamander population experiencing the decline during 2010. For comparison, skin swabs were collected from 51 clinically healthy fire salamanders from a population without a history of decline (N50°57′13″; E3°43′15″, Merelbeke, Belgium). DNA from the swabs was extracted in 100 μL PrepMan Ultra (Applied Biosystems) (8). Samples were examined for the presence of DNA of B. dendrobatidis using qPCR and for the presence of DNA of B. salamandrivorans using the PCR described above.
Phylogeny. In addition to the unique chytrid fungus, our taxon sampling consisted of three B. dendrobatidis strains and 27 species representing a broad evolutionary range of Chytridiomycota. In addition, Rozella allomycis and two Blastocladiomycota (Allomyces arbuscula and Catenaria anguillulae) were used as outgroup taxa. Alignment was done with ClustalX 2.0.10 (24), and ambiguously aligned fragments were excluded for further analysis, resulting in a 1,513-bp reliably aligned data matrix. Maximum parsimony (MP) and maximum likelihood (ML) analyses were performed using PAUP* 4.0b10 (25). Heuristic MP searches were executed in 10,000 replicates, with all characters unordered and equally weighted, and using tree bisection reconnection (TBR) branch swapping. The strict consensus tree of 81 equally most parsimonious trees (tree length = 1,471) supported the (B. dendrobatidis, B. salamandrivorans) sister relationship and received an MP bootstrap support of 100. Bayesian and likelihood analyses were performed with the GTR + G + I model of DNA substitution. For the likelihood analyses, heuristic searches were performed with substitution rates, γ-shape parameter, and proportion of invariable sites estimated from neighbor joining trees. These parameters were reestimated from the best ML tree found thus far, and the tree was submitted to additional rounds of TBR swapping; this procedure was repeated several times. These maximum likelihood analyses resulted in a single best tree [−ln L = 9,562.04266; pinvar = 0.301311; shape parameter α= 0.60887]. ML bootstrapping was done in 1,000 replicates with fixed parameters.
Bayesian analyses were done with MrBayes 3.1.2 (26). Two runs of four Markov chain Monte Carlo (MCMC) chains each were executed in parallel for 5,000,000 generations, with a sampling interval of 500 generations and a burnin corresponding to the first 1,000,000 generations. Posterior probabilities for clades were obtained by combining the post–burn-in trees from parallel runs in a single consensus tree. Convergence of the parallel runs was confirmed by split frequency SDs (<0.01) and potential scale reduction factors (approximating 1.0) for all model parameters.
ACKNOWLEDGMENTS. The technical assistance of M. Claeys, M. Couvreur, and C. Adriaensen is appreciated. We thank Dr. J. Z. Euzeby for his kindness in helping with the Latin for the species name. We thank the editor and two anonymous reviewers for their constructive comments, which improved the manuscript. I. Van Bocxlaer assisted with phylogenetic analyses. M.B. was supported by a Dehousse grant provided by the Royal Zoological Society of Antwerp. F.B. was supported by European Research Council Starting Grant 204509 [project Tracing Antimicrobial Peptides and Pheromones in the Amphibian Skin (TAPAS)]. M.C.F. was supported by the Biodiversa project Risk Assessment of Chytridiomycosis to European amphibian Biodiversity (RACE).
- Mendelson JR III, et al. (2006) Biodiversity. Confronting amphibian declines and extinctions. Science 313(5783):48.
- Crawford AJ, Lips KR, Bermingham E (2010) Epidemic disease decimates amphibianabundance, species diversity, and evolutionary history in the highlands of central
Panama. Proc Natl Acad Sci USA 107(31):13777–13782.
- Fisher MC, et al. (2012) Emerging fungal threats to animal, plant and ecosystemhealth. Nature 484(7393):186–194.
- Caruso NM, Lips KR (2013) Truly enigmatic declines in terrestrial salamander populations in Great Smoky Mountains National Park. Divers Distrib 19(1):38–48.
- Spitzen-van der Sluijs A, et al. (2013) Enigmatic decline drives Salamandra salamandra to the edge of extinction in The Netherlands. Amphib-reptil 34(2):233–239.
- Rosenblum EB, et al. (2013) Complex history of the amphibian-killing chytrid fungusrevealed with genome resequencing data. Proc Natl Acad Sci USA 110(23):9385–9390.
- Longcore JE, Pessier AP, Nichols DK (1999) Batrachochytrium dendrobatidis gen. et sp. nov., a chytrid pathogenic to amphibians. Mycologia 91(2):219–227.
- Hyatt AD, et al. (2007) Diagnostic assays and sampling protocols for the detection of
Batrachochytrium dendrobatidis. Dis Aquat Organ 73(3):175–192.
- Annis SL, Dastoor FP, Ziel H, Daszak P, Longcore JE (2004) A DNA-based assay identifies Batrachochytrium dendrobatidis in amphibians. J Wildl Dis 40(3):420–428.
4 of 5 | www.pnas.org/cgi/doi/10.1073/pnas.1307356110 Martel et al.
- Boyle DG, Boyle DB, Olsen V, Morgan JAT, Hyatt AD (2004) Rapid quantitative detection of chytridiomycosis (Batrachochytrium dendrobatidis) in amphibian samples using real-time Taqman PCR assay. Dis Aquat Organ 60(2):141–148.
- Doszpoly A, et al. (2008) Molecular confirmation of a new herpesvirus from catfish (Ameiurus melas) by testing the performance of a novel PCR method, designed totarget the DNA polymerase gene of alloherpesviruses. Arch Virol 153(11):2123–2127.
- Wellehan JFX, et al. (2004) Detection and analysis of six lizard adenoviruses by consensus primer PCR provides further evidence of a reptilian origin for the atadenoviruses. J Virol 78(23):13366–13369.
- Mao J, Hedrick RP, Chinchar VG (1997) Molecular characterization, sequence analysis,and taxonomic position of newly isolated fish iridoviruses. Virology 229(1):212–220.
- Martel A, et al. (2012) Novel Chlamydiaceae disease in captive salamanders. Emerg Infect Dis 18(6):1020–1022.
- Berger L, Hyatt AD, Speare R, Longcore JE (2005) Life cycle stages of the amphibianchytrid Batrachochytrium dendrobatidis. Dis Aquat Organ 68(1):51–63.
- Bosch J, Martinez-Solano I, Garcia-Paris M (2001) Evidence of a chytrid fungus infection involved in the decline of the common midwife toad (Alytes obstetricans) in protected areas of central Spain. Biol Conserv 97(3):331–337.
- Walker AF, et al. (2010) Factors driving pathogenicity versus prevalence of the amphibian pathogen Batrachochytrium dendrobatidis and chytridiomycosis in Iberia. Ecol Lett 13:372–382.
- Farrer RA, et al. (2011) Multiple emergences of genetically diverse amphibian-infectingchytrids include a globalized hypervirulent recombinant lineage. Proc Natl Acad Sci USA 108(46):18732–18736.
Martel et al.
- Olson DH, et al.; Bd Mapping Group (2013) Mapping the global emergence of Batrachochytrium dendrobatidis, the amphibian chytrid fungus. PLoS ONE 8(2): e56802.
- Boyle DG, et al. (2003) Cryo-archiving of Batrachochytrium dendrobatidis and other chytridiomycetes. Dis Aquat Organ 56(1):59–64.
- White TJ, Burns T, Lee S, Taylor J (1990) PCR Protocols: A Guide to Methods and Applications, eds Innis MA, Gelfand DH, Sninsky JJ, White TJ (Academic Press, San
Diego), pp 315–322.
- Garmyn A, et al. (2012) Waterfowl: Potential environmental reservoirs of the chytridfungus Batrachochytrium dendrobatidis. PLoS ONE 7(4):e35038.
- Lechter PM, Powell MJ (2005) Kappamyces, a new genus in the Chytridiales (Chytridiomycota). Nova Hedwigia 80(1-2):115–133.
- Thompson JD, Gibson TJ, Higgins DG (2002) Multiple sequence alignment usingClustalW and ClustalX. Current Protocols in Bioinformatics, eds Baxevanis AD,
Pearson WR, Stein LD, Stormo GD, Yates III JR (John Wiley & Sons, New York), pp
2.3.1-2.3.22.
- Swofford DL (2002) PAUP* 4.0 Phylogenetic Analysis Using Parsimony (*and Other
Methods) (Sinauer Associates, Sunderland, MA).
- Ronquist F, Huelsenbeck JP (2003) MrBayes 3: Bayesian phylogenetic inference undermixed models. Bioinformatics 19(12):1572–1574.
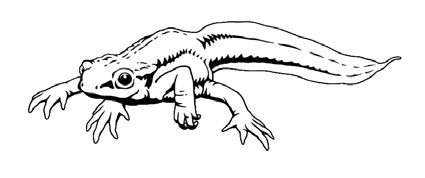
Actueel
Salamanders nog actief door zachte herfst
Het aanhoudend zachte weer zorgt ervoor dat veel salamanders hun winterslaap uitstellen. Vooral op vochtige avonden zijn ze nog te…
Japan ontdekt exemplaar van ’s werelds grootste bedreigde amfibie
Onderzoekers van de Kyoto Universiteit hebben recent de aanwezigheid van de Chinese reuzensalamander (Andrias davidianus), de grootste levende amfibie ter…
De Bedreigde Bandsalamander: Klimaatverandering en Bescherming
Dr. Uwe Gerlach, een expert in amfibieën uit Hattersheim, heeft een fascinerend nieuw boek uitgebracht over de bandsalamander (Ommatotriton ophryticus),…